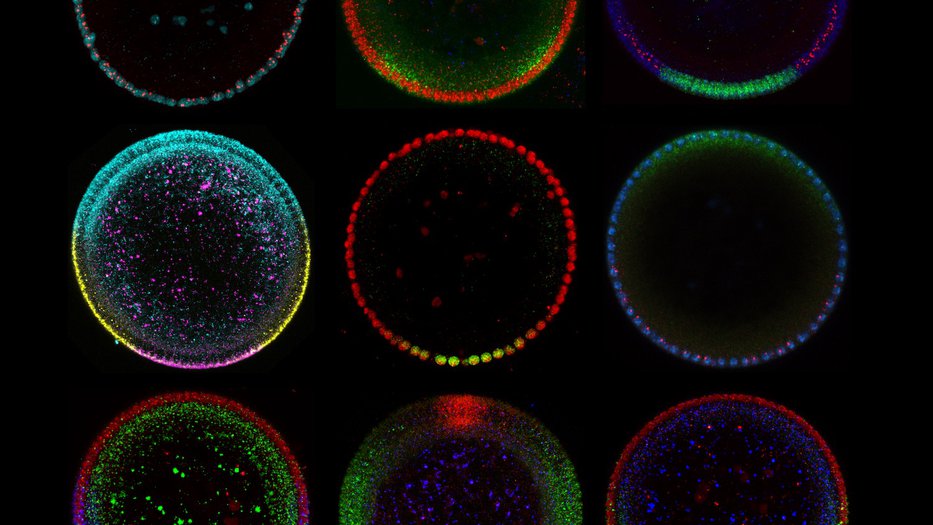
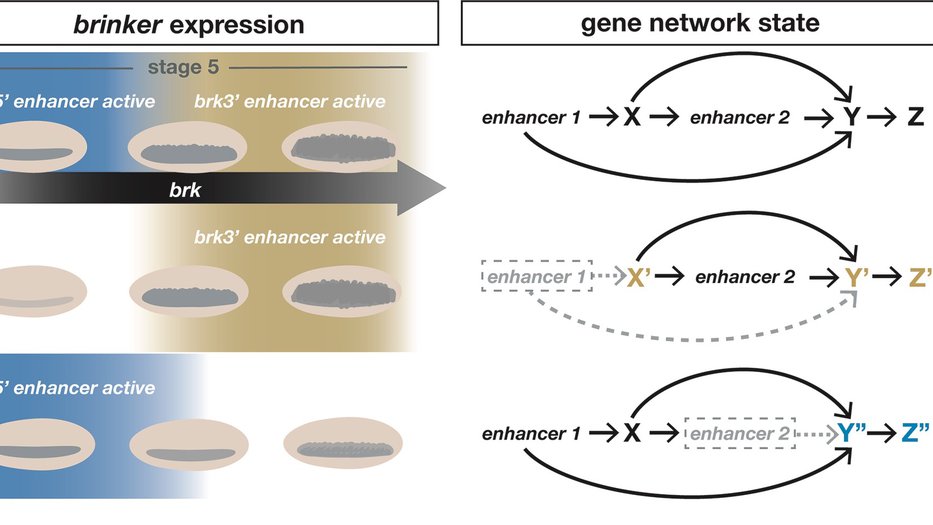
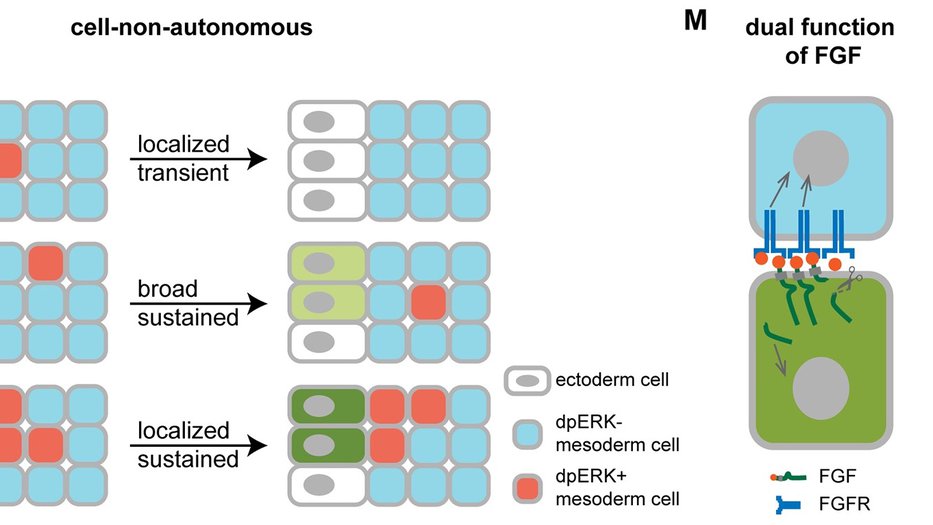
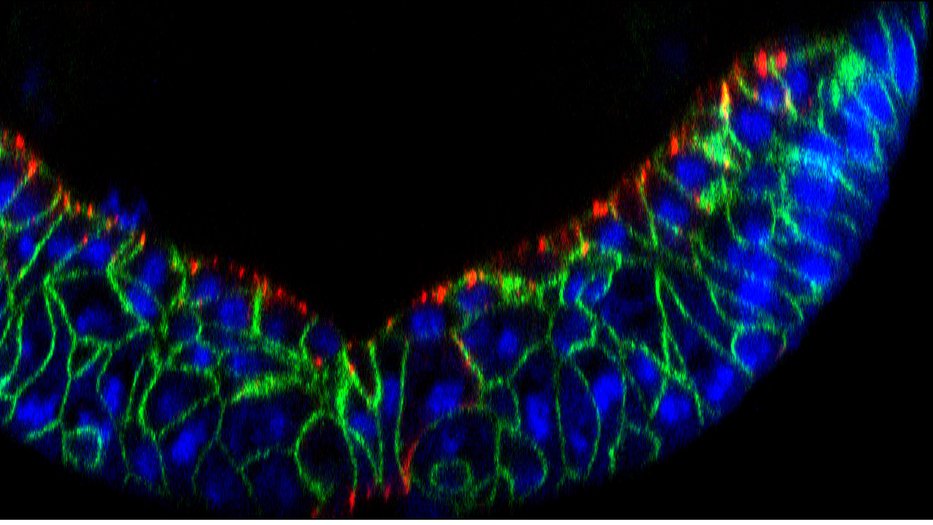
I. Embryonic Gene Expression: what controls timing?
We aim to expand understanding of the Dorsal-Ventral (DV) patterning gene-regulatory network (GRN) acting in the early Drosophila embryo: a developmental system, which uses morphogens to support patterning and undergoes rapid development. Our goal is to integrate spatiotemporal information into the DV patterning GRN with the objective of obtaining additional insights into the roles of transcription factor and target gene dynamics (see Reeves, Trisnadi et al. Dev Cell 2012). Recently, we have determined that broadly expressed repressors can influence when genes are allowed to turn on in embryos (see Koromila & Stathopoulos, Cell Rep 2019). In particular, they are important at the maternal-to-zygotic (MZT) transition. In addition, there are a number of pioneer activators that help to move nucleosomes, making the chromatin accessible to transcription factors including the pivotal activator Dorsal. We are interested in late-acting pioneers that regulate the MZT including regulating expression of genes pivotal for the onset of gastrulation and segmentation (see Koromila et al. eLife 2020). Many studies of patterning in the Drosophila embryo have focused on regulation of spatial outputs of gene expression. However, our studies are currently focused on how timing of expression is controlled, as this is certainly an equally important variable that embryos must regulate for proper development.
2. Enhancer Action: are there air-traffic controllers?
Many genes are pervasively expressed throughout development and exhibit changes of expression in a stage-specific manner. It is appreciated that different cis-regulatory modules (CRMs), also known as enhancers, act to control dynamic expression; however, not much is known about how enhancer order of action is regulated. Using the Drosophila embryo as a model system, we have the exceptional opportunity to investigate how enhancers support spatiotemporally-regulated gene expression during animal development. Current experiments focus on understanding how enhancer order of action is controlled. In particular, we are investigating whether promoter proximal elements work to balance enhancer input. This is important for regulating activation, for example, to control spatial outputs as well as levels of expression. The brinker gene locus is particularly well-suited to provide insight into the regulation of temporal gene expression. Our preliminary data show that the sequence just upstream of the brk minimal promoter contributes to sequential, coordinate action of CRMs controlling expression in the early embryo (see Dunipace et al., Dev Cell 2013). However, how this promoter proximal sequence as well as chromatin conformation supports timing of CRMs acting in series is not yet understood, and will be investigated. In addition, a necessary technical advance for analysis of dynamic developmental systems is analysis of chromatin conformation on a cell by cell basis, which will support studies of when and how particular CRMs interact with the promoter with temporal and spatial resolution. To this end, we are developing various technologies to acquire information about chromatin conformation and the Drosophila embryo is our test tube! We are also looking broadly at the regulation of gene expression over time and how enhancer action is regulated to effect change.
3. Fibroblast Growth Factors: do reverse signals exist?
Fibroblast growth factor (FGF) signaling impacts a number of different cellular functions important for supporting embryonic development. FGF ligands are polypeptide growth factors that bind to cell surface fibroblast growth factor receptors (FGFRs). These receptor ligands trigger tyrosine kinase activity associated with the intracellular domains of their receptors, and thereby activate signaling responses within cells. In early embryos, FGF signaling controls mesoderm induction and patterning, cell growth, migration, and differentiation; while later functions include organ formation and maintenance, neuronal differentiation and survival, wound healing, and malignant transformation. In vertebrates, nevertheless, the system remains quite complex with over 120 FGF-FGFR combinations possible. Studies in Drosophila have yielded valuable insights into the functions of many signaling pathways during development, as this system is amenable to molecular and genetic techniques as well as to live in vivo imaging. Drosophila, with only three FGF-FGFR combinations acting, offers a simpler system to study FGF signaling.
In a recent study, we identified structural features providing this information for one ligand of the FGF family named Pyramus (Pyr) (see Stepanik, Sun et al. Curr Biol 2020). These features control the dynamics of how the Pyramus signal is sent, thereby expanding the range of information that can be transferred from Pyramus to its receptor. One feature, a transmembrane domain, acts to tether the signaling portion of Pyramus to its cell of origin, thereby limiting how far the signal can travel. The second feature, known as a degron, leads to destruction of Pyramus when it is attached, and therefore strongly limits the amount of Pyramus produced until it is removed. This is the first study to identify these structural features in any FGF ligand (22 FGFs are present in humans alone). Importantly, this study demonstrates how these features affect the signaling properties of Pyramus as it is being utilized during development, by providing a local, concentrated signal (via the tether), and a signal that is sent in a precise direction (via the degron) at a precise time, which is especially critical when building complex three-dimensional tissues in an organism.
4. Cell Migration in Making Tissues: killing cells that are misdirected.
Cell migration is a very influential process during embryonic development as it results in rearrangement of cells from one part of the embryo to another, effectively controlling cell-cell interactions to drive cell differentiation and organogenesis. The shape of most complex organ systems arises from the directed migration of cohesive groups of cells. Therefore, cell migration must be regulated temporally and spatially for organisms to develop properly. The overlying goal of our research objective is to provide insight into how cells within a migrating groups sense their environment and how this contributes to their collective movement. We study the movement of mesoderm cells during gastrulation (see Sun and Stathopoulos, Dev 2018) and at later stages mesoderm cells which are precursors of longitudinal muscles (see Macabenta and Stathopoulos, Dev 2019). Caudal visceral mesoderm (CVM) cells exhibit directed cell migration as two distinct groups on either side of the body, from the posterior-most position of the embryo toward the anterior. The cells undergo the longest migration in all of Drosophila embryogenesis, but little is understood about how they are directed along their course. The migration ensues over six hours and is necessary to position CVM cells along the entire length of the developing gut. At the end of their migration, CVM cells fuse with fusion-competent myoblasts to form the longitudinal muscles which ensheath the gut. Curiously, if these migrating cells move off track they undergo apoptosis. We are interested in understanding the signals that guide migration of cells as well as the mechanism by which cells off-track are killed off.